Dr. Walt Brown's Hydroplate Theory
The hydroplate theory is an alternate explanation of both the events of the Noahic flood, the present-day geological features of the world, and the actual mechanisms that operated then and continue to do so now. It directly challenges the current plate tectonics model of large-scale geology, and it suggests a major revamping of the geological events associated with the flood that God sent upon the world in light of a hard-line exegetical approach to the text of Genesis.
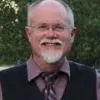
- Martin G. Selbrede
[Note by the compiler: After a half dozen editions, Dr. Walt Brown’s seminal text, In The Beginning: Compelling Evidence for Creation and the Flood has developed into a mature exposition of an important new approach to the geological sciences. This overview is intended for readers not yet familiar with Dr. Brown’s fresh and tightly-argued rethinking of the proper application of Scripture to geology. Although it diverges significantly from the work of other creationists working in the field, Dr. Brown’s theory deserves both respect and a full hearing based on its considerable merits. Inasmuch as Chalcedon’s commitment to creation science is long-standing — e.g., the inaugural edition of the Journal of Christian Reconstruction was devoted to the topic — it is hoped that a larger audience for these important ideas will be gained by their inclusion in the Report. We thank Dr. Brown for the opportunity to present his ideas to a new audience. — MGS.]
The Hydroplate Theory: A Brief Overview
The hydroplate theory is an alternate explanation of both the events of the Noahic flood, the present-day geological features of the world, and the actual mechanisms that operated then and continue to do so now. It directly challenges the current plate tectonics model of large-scale geology, and it suggests a major revamping of the geological events associated with the flood that God sent upon the world in light of a hard-line exegetical approach to the text of Genesis. It represents, then, a serious attempt at reconstructing the science of geology from the ground up.
Assumptions Undergirding the Hydroplate Theory
There are three assumptions upon which the hydroplate theory is built:
(1) Europe, Asia, Africa, and the Americas were joined across what is now the Atlantic Ocean, in the position shown in Figure 1 below. The fitting of the continents is not the conventional one, which requires that serious distortions be imposed on the pieces being forced to match up edge-to-edge. Conventional theory, as represented by Edward Bullard’s model, requires shrinking Africa by 40%, removing Central America, Southern Mexico, and the Caribbean Islands, rotating Europe counterclockwise while rotating Africa clockwise, and rotating all continents relative to one another, and even the “fit” resulting after all these machinations is poor, as shown in Figure 2 below. The hydroplate model does not try to fit existing coastlines together in a jigsaw puzzle, but utilizes the Mid-Atlantic Ridge as the correct “edge” to be fitted: this results in the best possible fit of the continents.
(2) Ten miles below the pre-Flood Earth’s surface were interconnected chambers of subterranean water — containing roughly half the liquid volume of today’s oceans. These chambers formed a thin, spherical shell of water with a mean thickness of 5/8 of a mile. This answers to the Biblical “waters of the deep” that burst open during the Noahic Flood. These waters contained enormous amounts of dissolved gases and minerals, particularly salt (NaCl) and carbon dioxide (CO2). A layer of basalt was situated between these waters and the Earth’s upper mantle.
(3) The final assumption of the hydroplate theory is that the pressure in the layer of subterranean water was increasing.
18 Geological Features in Search of a Doctrine
There are 18 distinct geological features that cannot be satisfactorily explained by current geological theory, and are accordingly the focus of continuing controversy.
(1) The Mid-Oceanic Ridge, discovered in the 1950s, is a mountain range 46,000 miles long that wraps around the Earth — on the ocean floor. It is formed of basalt, unlike almost all other mountains. The portion running down the center of the Atlantic Ocean, called the Mid-Atlantic Ridge, will be our primary focus. The explanations offered by plate tectonic theory will be shown to be less than satisfactory, whereas the hydroplate theory yields an explanation consistent with the actual features of the ridge.
(2) Continental shelves extend outward from the continents, sometimes for considerable distances, prior to plunging downward into deep sea regions. The boundary is considered to be halfway down the continental slope.
(3) Ocean trenches are long, narrow depressions on the ocean floor. Plate tectonics, which proposes that the earth’s crust is composed of roughly a dozen 30-mile-thick plates upon which the continents and oceans rest, treats these trenches as points where a moving plate dives down into the Earth’s mantle, a process called subduction. What pushes these 30-mile-thick plates down at such a steep angle, with frictional forces exceeding the strength of rock? Why do seismic reflection profiles show no distortion of the horizontal sedimentary layers in trenches, if they are the point where the proposed plates dive down into the mantle?
(4) Seamounts (submarine volcanos) litter the Pacific floor, some being almost as tall as Mt. Everest — however, there are few seamounts in the Atlantic. If one plate dives beneath another, as modern theory teaches, why aren’t seamounts scraped off the top of the descending plate? Hundreds of flat-topped seamounts, called tablemounts, are 3000-6000 feet below sea level. Apparently, wave action planed off their tops. Either sea level was once much lower, or ocean floors were higher, or both — each possibility raises new and difficult questions.
(5) Plate tectonic theory claims that earthquakes occur when plates rub against each other, temporarily lock, and then periodically jerk loose. Why are some earthquakes, many quite powerful, far from plate boundaries? Why do earthquakes occur when water is forced into the ground, after large water reservoirs are built and filled?
(6) Plate tectonic theory gained acceptance when an important discovery of the 1960s was misinterpreted. People were told that paralleling the Mid-Oceanic Ridge are bands of ocean floor that have a reversed magnetic orientation. At a few places, the pattern of “reversals” on one side is almost a mirror image of those on the other side. This suggested that the magnetic poles of the earth reversed in the distant past, and that molten rock spreading away from the ridge solidified, took on the earth’s current magnetic orientation, and moved outward from the ridge like a conveyor belt.
This story is inaccurate. There are no magnetic reversals on the ocean floor, and no compass would reverse direction if brought near the supposedly “reversed” bands in the Atlantic. There is, however, a fluctuation in magnetic intensity (see Figure 3 below). Someone merely drew a dashed line through these fluctuations and labeled everything below this average intensity a “reversal.” The false but widespread notion is that these deviations from the average represent the magnetic field from millions of years ago. This faulty understanding has prevented the formulation of a better explanation for these magnetic anomalies, including the added consideration that many of these bands are not parallel to the ridge, but perpendicular to it and lined up with fracture zones, contrary to plate tectonic predictions.
(7) Submarine canyons are often much larger than those found on the continents. One is three times deeper than the Grand Canyon, another is ten times longer (2,300 miles). Many of these V-shaped canyons are extensions of major rivers. How did they form? What force could gouge out a network of canyons 15,000 feet below sea level?
(8) There are surprisingly large amounts of coal in Antarctica, as well as fossilized tree trunks of considerable size. Was it once warm enough for trees to grow in Antarctica? If it was, how could so much vegetation grow where it is night 6 months of the year?
(9) How does an ice age begin or end? As glaciers expand, they reflect more of the sun’s radiation away from the earth, lowering global temperatures and causing even further glacier growth: a cycle that should continue until the entire globe is frozen. Conversely, if glaciers diminish, as they have in recent years, the earth should reflect less heat, warm up, and melt all glaciers forever.
(10) Some fleshy remains of about 50 mammoths and rhinoceroses have been found frozen and buried in Alaska and Siberia. One mammoth still had identifiable food in its mouth and stomach. To reproduce this result today, one would have to suddenly push a well-fed elephant (dead or alive) into a very large freezer and turn the thermostat to –150°F. This alone would prevent residual heat and gastric acid from destroying the food in the stomach, as well as explain why food would still be in the creature’s mouth.
Today the average January temperature in Siberia is –30°F: how did huge herds of these mammoths thrive at these temperatures, let alone find water to drink? Or were the Arctic regions much warmer in the past?
(11) How did the mountains form? Major mountains are usually crumpled like an accordion (see Figure 4). What force could push a long, thick slab of rock and cause it to buckle and sometimes fold back on itself without crushing the end being pushed? Even if the sediments were squeezed and folded prior to hardening, what squeezed them?
(12) Large blocks of rock called overthrusts present a similar problem: such blocks are thought to have slid over other rock for many miles. Why overthrusts occur has never been adequately explained. Anything pushing a large slab of rock with enough force to overcome frictional resistance would crush the slab before it would move. Although appeal is sometimes made to the pore pressure of water in the rocks providing the requisite lubrication to enable the sliding to take place on a downhill slope, not enough water resides in rocks today to make this possible, and over-thrusted blocks are not on slopes.
(13) Erupting lava usually exceeds 1800°F. Where does it come from and why is it so hot? The standard explanation is that magma originates in hot pockets called magma chambers at depths of about 60 miles. But how could magma escape to the surface? At depths greater than 4 or 5 miles, the pressure is so great that all empty channels through which magma might rise should be squeezed shut. Even if a crack could open, the magma must rise through colder rock — the magma would tend to solidify and plug up the crack.
The two deepest holes in the world are on the Kola Peninsula in northern Russia and in Germany’s northeastern Bavaria. Drilled to depths of 7.5 and 5.6 miles respectively, neither hole reached the basalt that underlies the granite continents. Deep in the Russian hole, to everyone’s surprise, was hot, flowing, mineralized water (including salt water) encased in crushed granite. Why was the granite crushed? In the German hole, the drill encountered salt-water-filled cracks throughout the lower few miles, with salt concentrations twice that of sea water. Surface water cannot migrate below about 5 miles because the weight of the overlying rock squeezes shut even microscopic flow channels. Although geologists are mystified by the presence of this deep salt water, the hydroplate theory resolves the mystery.
(14) Had the earth ever been molten, denser materials would have sunk toward the earth’s center, and lighter ones floated to the surface. One should not find dense metals like gold at the earth’s surface. No suggested transport mechanism satisfies all the requirements of this problem (e.g., volcanos transport material to the surface, but gold is not concentrated around volcanos). Even granite, the basic continental rock, is a mixture of many minerals with varying densities. If one melted granite and slowly cooled the liquid, the granite would not reform. Instead, it would become a layer cake of minerals sorted vertically by density. In other words, the earth’s crust appears to have never been molten.
Geothermal heat measurements vary widely across the globe, and tend to challenge both the “molten earth” model and the idea that billions of years of cooling have transpired. What, then is the source of geothermal heat and why do the measurements associated with it (“temperature gradients”) fluctuate so widely?
(15) Limestone (calcium carbonate, CaCO3) presents a challenge to modern geology: there’s too much of it based on the processes currently proposed to synthesize it. Most limestone is in extensive layers, tens of thousands of square miles in area and hundreds of feet thick, much of it quite pure. Under the Bahamas, the limestone is more than 3 miles thick! The presence of pure limestone, without the impurities that tend to drift in, argue for its rapid burial. Today, limestone forms either by precipitating out of sea water or by organisms taking it out of sea water to produce shells. In either case, oceans supply limestone sediments. The oceans already have as much limestone in them as they can possibly hold. Therefore, where did all the limestone come from, especially its calcium and carbon, which are relatively rare outside of limestone?
(16) Metamorphic rock presents enigmas of its own. Marble, a metamorphic rock, forms when limestone is heated beyond 1600°F and squeezed at a confining pressure corresponding to the weight of a 23-mile high column of rock. Such metamorphic rocks are formed in the presence of water, often flowing water. What could account for the extreme pressure, temperature, and abundance of water?
Mt. Everest being only 5.5 miles high, it is difficult to imagine mountains 23 miles high, but modern geologists who think in terms of millions of years don’t see any difficulties here: the metamorphic rock is slowly transported from many miles under the surface up to where we can find it. However, this explanation ignores the water issue: surface water cannot seep any lower than about 5 miles, and even at a 5 mile depth it does not flow. Where did the flowing water come from at the requisite 23-mile depth?
(17) Plateaus are relatively flat regions of large area that have been uplifted more than 500 feet relative to their surroundings. The standard model cannot explain their formation — the only explanation offered thus far invokes slow moving “convection currents” in solid rock some 30 miles below the surface sweeping enormous amounts of light rock from an unknown location and depositing it underneath the plateau. The Colorado plateau would require 2,500,000 cubic miles of granite to have been so transported, while the Tibetan plateaus would require 25,000,000 cubic miles of granite to have been swept under the region. In both instances, it is difficult to understand how this process deposited the granite in so uniform a layer, yielding a flat plateau of considerable extension (750,000 square miles of plateau in Tibet, for example). The source for this granite is even more troubling: the place from which this light rock originated should have been turned into an enormous geological depression, but no such predicted features have ever been observed on the earth.
(18) Thick layers of salt are buried up to several miles below the earth’s surface, sometimes in layers 100,000 square miles in area and a mile in thickness. Large salt deposits are not being laid down today. What concentrated so much salt? Sometimes a salt layer bulges up several miles, like a big underground bubble, to form a salt dome. Surprising large salt deposits lie under the Mediterranean; some have estimated that the Mediterranean must have evaporated 8-10 times to deposit so much salt. Although this estimate is probably low, the more damaging question is why each alleged refilling of the Mediterranean didn’t dissolve the salt residue left from the previous evaporation cycle.
Hydroplate Theory: Initial Proposals
The hydroplate theory proposes that the continents were once in the position shown in Figure 1, and that they were connected by rock that was rapidly eroded and transported worldwide by erupting subterranean water. Most of the earth’s sediments were formed from this eroded rock, which was once situated in the space between the continents in Figure 1. The continents quickly slide (rapid continental drift) east and west from what is now the Mid-Atlantic Ridge and came to rest in their present positions.
Evaluation Criteria for Geologic Models
Three criteria should govern the evaluation of any proposal in the hard sciences: process, parsimony, and prediction. A proposed process may have a host of collateral implications and consequences: if these are absent, or contradicted by the data, the initial proposal is thereby weakened. A proposal should invoke the principle of parsimony: the minimal use of assumptions (particularly ad hoc assumptions to “save the theory”). A scientific model should make confirmable predictions to provide a means by which it may either be strengthened or falsified in light of an ever-increasing amount of physical data.
Inasmuch as the event being described by the hydroplate theory is unrepeatable, it is necessary that certain assumptions be invoked (the three laid out at the beginning of this discussion). From that foundation, the events as detailed within the theory follow in logical succession and are described below.
The Hydroplate Theory: Events
The Rupture Phase of the Noahic flood began as increasing pressure in the subterranean water stretched the overlying crust, just as a balloon stretches when the pressure inside it increases. Eventually, this shell of rock reached its failure point. Failure began with a microscopic crack. Stress concentrations at both ends of the crack resulted in its rapid propagation at about 2 miles per second, nearly the velocity of sound in rock. The crack followed the path of least resistance, generally along a great-circle path. The ends of the crack, traveling in opposite directions, circled the earth in several hours. The initial stresses were largely relieved when one end of the crack ran into the path left by the other end. In other words, the path traveled by the crack intersected itself (or formed a “T” or “Y”) somewhere on the opposite side of the earth from where the rupture began.
As the crack raced around the earth, the 10-mile-thick “roof” of overlying rock opened like a rip in a tightly stretched cloth. The pressure in the subterranean chamber immediately beneath the rupture suddenly dropped to almost atmospheric pressure, causing water to explode with great violence out of the ten-mile-deep “slit” that wrapped around the earth like the seam of a baseball.
All along this globe-circling rupture, a fountain of water jetted supersonically into and above the atmosphere (Figure 5 below). The water fragmented into an “ocean” of droplets that fell to the earth great distances away. This produced torrential rains such as the earth has never experienced. Some jetting water rose above the atmosphere where the droplets froze. Huge masses of extremely cold, muddy “hail” fell at certain locations where it buried, suffocated, and froze many animals, including some mammoths.
The Flood Phase ensued as the extreme force of the 46,000-mile-long sheet of upward-jetting water rapidly eroded both sides of the crack. Eroded particles (or sediments) were swept up in the waters that gushed out from the rupture, giving the water a thick, muddy consistency. These sediments settled out over the earth’s surface in days, trapping and burying many plants and animals, beginning the process of forming most of the world’s fossils.
The rising flood waters eventually blanketed the water jetting from the rupture, although water still surged out of the rupture. Global flooding occurred over the earth’s relatively smooth topography, since today’s major mountains had not yet formed.
The temperature of the escaping subterranean waters increased by about 100°F as they were forced from the high pressure chamber. The hot water, being less dense, rose to the surface of the flood waters. There, high evaporation occurred, increasing the salt content of the remaining water. Once supersaturated, salts precipitated into thick, pasty layers. Later, the pasty (low density) salt was blanketed by denser sediments, creating an unstable arrangement of heavy material over lighter material. A slight jiggle will cause a plume of the lighter layer below to flow up through the denser layer above. In the case of salt, that plume is called a salt dome.
The pressure of the water decreased as it rose out of the subterranean chamber. Since high pressure liquids hold more dissolved gases than low pressure liquids, gases bubbled out of the escaping waters. This process occurs when a can of carbonated beverage is opened, releasing bubbles of dissolved carbon dioxide. From the subterranean waters, the most significant gas was carbon dioxide. About 35% of the sediments were eroded from the basalt below the escaping water. Up to 6% of basalt is calcium by weight. Calcium ions in the escaping water, along with dissolved carbon dioxide gas (carbonic acid) caused vast sheets of limestone (CaCO3) to precipitate as the pressure dropped.
The flooding uprooted most of the earth’s abundant vegetation. Much of it was transported by the flood’s currents to regions where it accumulated in great masses. Some vegetation even drifted to the South Pole. Later, during the continental drift phase, buried layers of vegetation were rapidly compressed and heated, precisely the conditions to form coal and oil. The flood phase ended with the continents near the positions shown in Figure 1 (viewed from space) and Figure 6 (viewed in cross-section).
The Rapid Continental Drift Phase develops as a consequence of the slight elasticity of compressed rock. The deeper the rock, the more tightly compressed is the “spring.” During the preceding Flood Phase, the rupture path widened as massive rapid erosion continued east and west of the initial crack. Eventually the eroded region was sufficiently wide that the compressed rock beneath the subterranean chamber was on the verge of springing upward. Centrifugal force is greatest at the equator, providing a slightly greater “outward tug” on the compressed rock where the rupture crossed the equator. The 46,000-mile-long rupture only crossed the equator at two places: one, in what is now the Pacific, and the other, in the Atlantic. However, the Atlantic location lies along the equator for 2,000 miles. Its length and location, then, caused the initial instability to occur there. As the ridge rose, it lifted adjacent material just enough to cause it to become unstable and also spring upward. This process continued all along the path of the rupture, forming the Mid-Oceanic Ridge. (See Figure 7 below for an illustration of the principle involved.) Also formed were fracture zones and the strange offsets the ridge makes along fracture zones. Soon afterward, the magnetic anomalies developed.
The ridge rose several miles and elevated the granite plates along the flanks of the ridge. As the plates rose, they began to slide downhill. The plates were well lubricated by subterranean water still escaping from beneath them. They slid east and west, because the Mid-Atlantic Ridge extends north and south.
Continental plates accelerated away from the segment of the Mid-Oceanic Ridge now called the Mid-Atlantic Ridge. As they did, the Atlantic Ocean basin opened up. Eventually the drifting (actually accelerating) continental plates (or hydroplates) ran into resistances of two types. The first happened as the water lubricant beneath each sliding plate was depleted. The second occurred when a plate collided with something. For example, India literally collided with Asia, and the western coast of North America collided with a rising portion of the Mid-Oceanic Ridge. As each massive hydroplate decelerated, it experienced a gigantic compression event — buckling, crushing, and thickening each plate.
Buckling occurred In the thinner portions of the hydroplates. Crushing and upward buckling formed major mountain ranges. As explained earlier, the forces for this dramatic event could not be applied to stationary (static) continents resting on other rock. The force was dynamic, produced by rapidly moving hydroplates riding on lubricating water that had not yet escaped from below them.
Naturally, the long axis of each buckled mountain and each trench was perpendicular to its hydroplates motion — or parallel to the portion of the Mid-Oceanic Ridge from which it slid. Thus, the Rocky Mountains, Appalachians, and Andes have a north-south orientation, The Himalayas have a northwest-to-southeast orientation because their hydroplate slide from the Mid-Indian Oceanic Ridge.
Friction at the base of skidding hydroplates generated immense heat, enough to melt rock and produce massive volumes of magma. In some regions, the high temperatures and pressures formed metamorphic rock. Where this heat was intense, rock melted. This high pressure magma squirted up through cracks between broken blocks, producing other metamorphic rocks. Sometimes it escaped to the earth’s surface, producing volcanic activity and “floods” of lava outpourings, such as we see on the Columbia and Deccan Plateaus. This was the beginning of the earth’s volcanic activity.
Other magma collected in pockets, now called magma chambers. The volcanic activity surrounding the Pacific Ocean, the so-called “ring of fire,” corresponds to the leading edges of the hydroplates where compression and crushing would have generally been the greatest. The heat remaining today is called geothermal heat.
As the continents rose out of the water, and mountains formed, some subterranean water also flowed up into the cracks in the crushed granite. This is what was encountered in the deep holes drilled in Russia and Germany. We can now understand why the salt concentration in these cracks was about twice that of sea water. The preflood seas, which had little dissolved salt, diluted by about half the equal volume of salty, subterranean water that gushed out during the flood. Salty water that did not escape, therefore, has twice the salt concentration of today’s oceans.
The Recovery Phase followed the compression event, which entailed the receding of the flood waters as the mountains were buckled and folded up from the leading edges of the sliding hydroplates.
Simultaneously, the violent force of the upward surging subterranean water was “choked off” as the plates settled onto the floor of the subterranean chamber. Without sinking hydroplates to produce the high pressure flow, water was no longer forced up through the rupture. Instead, the deep basins between the continents became reservoirs into which the flood waters returned. These deep reservoirs were initially part of the basalt floor of the subterranean chamber, 10.75 miles below the earth’s surface. Consequently, sea level immediately after the flood was several miles lower than it is today. This provided wide land bridges between all continents, facilitating the migration of animals and people for perhaps several centuries. Drainage of the flood waters down the steep continental eroded deep channels which today are called submarine canyons.
Hydroplates rested on some parts of this basalt floor, while water covered other portions. Since the thickened hydroplates applied greater pressure to the floor than did the water, the hydroplates depressed the basalt floor downward over the centuries. The material the sinking plates aced caused the deep ocean floor to rise. (Imagine a water bed suddenly covered by two adjacent plates. The denser plate will sink, lilting the other plate.)
As sea level rose in the centuries after the flood, animals were forced to higher ground and were sometimes isolated on islands far from our present continental boundaries. Classic examples of this are the finches and other animals Charles Darwin found on the Galapagos Islands, 650 miles off the coast of Ecuador. Today, those islands are the only visible remains of a drowned South American peninsula. Darwin believed the finches were blown there during a giant storm. (While some may believe that story, it also requires that both a male and female finch ended up on the same island, or at least one pregnant female.)
The more sediments continents carried and the thicker continents grew during the crushing of the compression event, the deeper they sank. This gave rise to changing depths of the crust-mantle interface called the Mohorovocic Discontinuity (or Moho for short). This explains why continental material is so different from oceanic material, and why the Moho is so deep beneath mountains and yet so shallow beneath the ocean floor.
Over the. centuries, new mountain ranges and thickened continental plates settled slowly to their equilibrium depth. Sinking mountains increased the pressure under the crust on both sides of mountain ranges. Consequently, weaker portions of the overlying crust fractured and rose, forming plateaus, even on the ocean floor. In other words, as continents and mountains sank, plateaus rose. This serves to explain the seemingly strange aspects of plateaus noted earlier. It also explains why plateaus are adjacent to major mountain ranges. The Tibetan Plateau is next to the most massive mountain range in the world — the Himalayas, while the Colorado Plateau is next to the Rocky Mountains and the Columbia Plateau next to the Cascades.
Drainage of the waters that covered the earth left every continental basin filled to the brim with water. Some of these postflood lakes lost more water by evaporation and seepage than they gained by rainfall and drainage from higher elevations. Consequently, they shrank over the centuries. A well-known example was former Lake Bonneville which became the Great Salt Lake.
Through rainfall and drainage from higher terrain, other lakes gained more water than they lost and thus overflowed their rims at the lowest point. The resulting erosion at that point on the rim allowed more water to flow over it. This eroded the cut in the rim even deeper and caused even more water to cut it faster. Thus, the downcutting process accelerated catastrophically. Eventually, the entire lake dumped through a deep slit which we today call a canyon. These waters emptied into the next lower basin, causing it to breach its rim and create another canyon, like falling dominoes. The most famous canyon of all. Grand Canyon, was caused primary by the dumping of what we will call Grand Lake. It occupied the southeast quarter of Utah, parts of northeastern Arizona, as well as small parts of Colorado and New Mexico. Grand Lake, standing at an elevation of 5,700 feet above today’s sea level, spilled over and quickly eroded its natural dam 22 miles southwest of what is now Page, Arizona. In doing so, the western boundary of former Hopi Lake (elevation 5,950 feet) was eroded, releasing waters that occupied the present valley of the Little Colorado River. In just a few weeks, more water was released over northern Arizona than is in all the Great Lakes combined.
With thousands of large, high lakes after the flood, and a lowered sea level, many other canyons were carved. Some are now covered by the raised ocean. It appears likely that (1) the Mediterranean “Lake” dumped into the lowered Atlantic Ocean and carved a canyon at the Strait of Gibraltar, (2) the Black Sea carved out the Bosporus and Dardanelles, and (3) “Lake California” filling the Great Central Valley of California carved a canyon (now largely filled with sediments) under what is now the Golden Gate bridge in San Francisco. PREDICTION 1: The crystalline rock under Gibraltar, the Bosporus and Dardanelles, and the Golden Gate bridge is eroded into a Y-shaped notch.
Shifts of mass upon the earth created stresses and ruptures throughout the solid earth. This was especially severe under the Pacific, since the major continental plates all moved toward it. In regions now occupied by ocean trenches, gravity-driven granite plates were warped downward by movements, throughout the inner earth toward the rising Atlantic floor on the opposite side of the earth.
Surrounding the Pacific is a region called “the ring of fire,” the highest concentration of volcanic activity on earth. However, within “the ring of fire,” hidden on the floor of the Pacific, is past volcanic activity and lava flows of a much greater magnitude. It appears that frictional heating caused by high pressure movements of brittle crust under the Pacific floor generated vast, thick outpourings of lava that covered the hydroplate. Thus, the floor of the western Pacific is littered with volcanic cones composed of minerals that are typically found in granite and basalt. Continental crust has been discovered under the floor of the northwestern Pacific. PREDICTION 2: A mile or so under the entire western Pacific floor will he found a granite hydroplate.
The beginning of earthquake activity also coincided with the end of the flood. Rock was buckled down into regions of higher temperature and pressure. Some minerals that compose a large fraction of the mantle undergo several types of phase transformation; that is, their atoms rearrange themselves into a denser packing arrangement when the temperature and pressure rise above certain thresholds. For example, olivine (a prominent mineral in the mantle) snaps into an atomic arrangement called spinel having about 10% less volume. The collapse begins at a microscopic point and creates a shock wave. A larger pocket of rock, that is already sufficiently heated, then exceeds its pressure threshold. The resulting implosion is a deep earthquake. Over the many centuries since this worldwide cataclysm, the downbuckled rock has slowly heated up, and it periodically implodes.
Figure 7a-c. Spring Analogy Relating to the Development of the Mid-Atlantic Ridge. The rocks represent the regions adjacent to the widening gap eroded by the escaping subterranean water.
The reverse process, sudden expansion, occurs at the uplifted Mid-Oceanic Ridge. There, some minerals slowly swell and rearrange themselves into a less dense packing arrangement. The swelling at the ridge and the shrinking at the trenches cause the skin, of the earth to slide in jerks along its “near-zero-shear-strength surface” 125 miles below the earth’s surface. Earthquakes also occur under hydroplates wherever there has been a large, vertical displacement.
Shallow earthquakes involve a different phenomenon. The following may explain what happens. Trapped, subterranean water, unable to escape during the flood, slowly seeps up through cracks and faults formed during the compression event. The higher this water migrates through crack, the greater its pressure is in comparison to the walls of the crack trying to contain it. This tends to spread the cracked rock and lengthen the crack. (This may explain why the ground often bulges slightly before an earthquake and water levels sometimes change in wells.) Stresses build up in the crust as the Mid-Oceanic Ridges swell and trenches contract. Once the compressive stress has risen enough, the cracks have grown enough, and the degree of frictional locking of cracked surfaces has diminished enough, sudden movement occurs. The water then acts as a lubricant. (This explains why frictional heat was not found along the San Andreas fault.) Sliding friction almost instantaneously heats the water, converts it to steam at an even higher pressure, and initiates a runaway process called a shallow earthquake. This movement of the remaining subterranean water produces imbalances and partial voids which trigger even deeper sudden movements. PREDICTION 3: Moderately deep holes, drilled along major faults in populated regions, will provide an easy escape for seeping, high pressure subterranean water near the hole. Shallow earthquake frequency in the region will diminish. Of course, stresses will continue to build up, but some of that energy will be dissipated by the flow of deep viscous rock. Bleeding off subsurface water will reduce the runaway effect caused by the frictional heating of the lubricating water. Sudden increases in the water’s depth in many of these holes may serve as a precursor to shallow earthquakes.
Frictional heating at the base of sliding hydroplates and in movements within the rising ocean floors produced warm oceans, high evaporation rates, and heavy cloud covet. The elevated continents, which required centuries to sink to their equilibrium level, were consequently colder than today. Volcanic debris and heavy cloud cover shielded the earth’s surface from much of the sun’s rays, producing the ultimate “nuclear winter.” At higher latitudes and elevations, such as the newly elevated and extremely high mountains, this combination of high precipitation and low temperatures produced very heavy snow falls — perhaps 100 times that of today. Large temperature differences, between the cold land and warm oceans generated high winds that rapidly transported moist air up onto the elevated, cool continents where heavy snowfall occurred, especially over glaciated areas. As snow depths increased, periodic and rapid movements of the glaciers occurred in “avalanche fashion.” During the summer months, rain fell instead of snow, causing the glaciers to partially melt and retreat, thus marking the end of that year’s “ice age.”
Many seamounts grew up to the surface of the lowered ocean, where their peaks were eroded and flattened by wave action. These flat-topped or truncated cones are now called tablemounts. Their eroded tops are several thousand feet below today’s sea level. Sea level continued to rise as glaciers melted and retreated to their present positions. Glacial retreat continues today.
The Significance of Liquefaction
Liquefaction is a poorly-understood phenomenon. We will first consider liquefaction on a small scale. Alter understanding why it occurs, we will see that a global flood would produce massive liquefaction on a worldwide scale. Finally, a review of other poorly-understood features in the earth’s crust will confirm that global liquefaction did occur.
Examples of Liquefaction
Quicksand is a simple example of liquefaction. Quicksand is sand up through which spring-fed water flows. The upward flowing water lifts the sand grains very slightly, surrounding each grain with a thin film of water. This cushioning gives quicksand, and other liquefied sediments, a spongy, fluid-like texture.
Contrary to popular belief, someone stepping into quicksand does not sink out of sight forever. They will quickly sink in — but only so far. They then will be lifted, or buoyed up, by a force equal to the weight of the sand and water displaced. The more they sink, the more they will be lifted. Quicksand’s buoyancy is almost twice that of water, because the weight of the displaced sand and water is twice that of water alone. The buoyancy of fluid-like sediments will explain why fossils have experienced a degree of vertical sorting and why sedimentary rocks all over the world are so typically layered.
Once we understand the mechanics of liquefaction, we can identify situations where liquefaction would have occurred massively and continuously for weeks or months — all over the earth.
Visualize a box filled with small rocks. Shaking the box will cause the rocks to settle into a denser packing arrangement. Repeat this thought experiment, only this time all the spaces between the rocks ate filled with water. As you shake the box and the rocks settle into a denser arrangement, water will be forced up to the top by the weight of the falling rocks. If the box is tall so that many rocks fall, the force of the rising water will increase, and the topmost rocks will be lifted by water pressure for as long as the water flows.
This is similar to an earthquake in a region having loose, water-saturated sediments. Once upward flowing water lifts the topmost sediments, the next level of sedimentary particles no longer has the weight of the topmost layers pressing down on them. This second layer can then be more easily lifted by the force of upward flowing water. This in turn unburdens the third layer of sediments, etc. The particles are no longer in solid-to-solid contact, but are now suspended in and lubricated by water, so they can slip by each other with ease.
Wave Loading: Three Examples
As you walk barefooted along the beach, each ocean wave comes in, and water rises from the bottom of your feet to your knees. When the wave recedes, the sand beneath your feet becomes very loose and mushy, causing your feet to sink in. This is a small example of liquefaction which everyone has experienced. At the height of each wave, water is forced down into the sand. As the wave returns to the ocean, the water forced into the sand gushes back out, lifting the topmost grains and forming a mushy mixture.
During storms, high waves have caused liquefaction on parts of the sea floor. This has resulted in the failure of pipelines buried offshore. As a large wave passes over a buried offshore pipe, the water pressure increases above it. This in turn forces more water into the porous sediments. As the wave peak passes and the trough approaches, the stored, high-pressure water in the sediments begins to flow upward. This lifts the sediments and causes liquefaction. The buried pipe, in floating upwards, breaks.
On November 18, 1929, an earthquake struck the continental slope off the coast of Newfoundland. Minutes later, transatlantic phone cables began breaking sequentially. The exact time and location of each break were recorded and are known. It was reported to have been a 65 mile-per-hour current of muddy water that snapped 12 cables in 28 places as it swept 400 miles down the continental slope from the earthquake’s epicenter. (This is known as the “turbidity current” explanation for the cable ruptures, a large area of study within geology.)
The problem with this alleged 65 mph muddy flow is that even the best nuclear-powered submarines cannot travel that fast, and that the average slope of the ocean floor in that area off the coast of Newfoundland is less than 2 degrees. Also, some broken cables were at a higher elevation than the ocean floor nearest to the earthquake. It seems more likely that a large wave (tsunami) radiated out from the epicenter. Liquefaction, occurring below the expanding wave, left segments of the transatlantic cables without support, causing them to snap.
The important fact to distill from all these examples is that liquefaction occurs whenever water is forced up through loose sediments with enough pressure to lift the topmost sedimentary particles.
Liquefaction During the Flood
The flooded earth would have had enormous, unimpeded waves, especially tidal waves caused by the gravitational attraction of the sun and moon. Today, most of the energy in tidal waves is dissipated as they reach coast lines, but a flooded earth would have no coastlines, so that much of the tidal energy would be carried around the earth to reinforce the next tidal wave. Under these conditions, tidal wave heights of almost a hundred feet have been simulated by computer. (Today the average amplitude is a mere 30 inches, with some notable exceptions due to bay shape.) At high tide during the flood, water would have been forced into the ocean floor by two mechanisms. First, water is slightly compressible.
At high tide, water in the saturated sediments below the wave is compressed like a spring. Second, at high tide, water is forced, not just down into the sediments below, but laterally through the sediments, in the direction of decreasing pressure. As the tidal wave diminishes, and the local pressure is reduced, that compressed water reemerges as upward flowing water.
Throughout the flood phase, a liquefaction cycle must have taken place every 12 hours and 25 minutes, the length of todays tidal cycle. Half the time, water would have been pushed down into the sediments, being stored for the other half-cycle, the discharge half, in which water would flow upward. Only during part of this discharge half would the waters upward velocity have been sufficient to cause liquefaction. When it did, many interesting things would happen. (See Fig. 9 in particular.)
Water flowing up through a bed of sediments with enough velocity will lift and support each sedimentary particle with water pressure. Rather than thinking of the water as flowing up through the sediments, we can think of the sediments as falling through a very long column of water. The slightest difference in a particle’s density, size, or shape will cause it to fall at a slightly different speed than an adjacent particle. Therefore, these particles are continually changing their relative positions until the water’s velocity or pressure drops below a certain value or until nearly identical particles are adjacent to each other and “fall” at the same speed. This provides sorting which accounts for the layering that is so typical of sedimentary rocks. Such sorting explains why several investigators have observed horizontal strata in large mud deposits from local floods. Liquefaction created the layering effect.
Using the apparatus shown in Figure 10, it is possible to illustrate key liquefaction principles. Each liquefaction cycle simulated by tilting the mechanism to force water to flow into the bottle containing various sediments caused the sediments to sort into clearly defined layers. The longer liquefaction is continued, the sharper the boundaries became between different sedimentary layers.
Another important phenomenon observed in this apparatus is called lensing. Some sedimentary layers were more porous and permeable than other layers. If water could flow more easily through a lower layer than it could through the layer immediately above it, a lens of water would accumulate at their interface. Water lenses were usually at small angles to the horizontal. In such lenses, the water always flowed uphill.
During the flood, liquefaction probably lasted for many hours twice a day. In a liquefaction column, many thick water lenses would have formed. Organisms would have floated up to the lens immediately above. Those of similar size, shape, and density (usually of the same species) would have been swept at similar rates along a nearly horizontal channel and spread out for many miles. Water’s buoyant force is much less than that of liquefied sediments, so water alone would have been less able to lift dead organisms into the denser sedimentary layer immediately above the lens.
Once the liquefaction phase of that cycle ended, the lens would disappear. The layers would settle tightly together, leaving fossils of one species spread over a wide surface which geologists would call a horizon. Thousands of years later, this would give most investigators the false impression that the species died long after the layers below it were deposited and long before the layers above it were laid down. When a layer with many fossils covered a vast area, it would be mistaken as an extinction event or, perhaps, as a boundary between geologic periods.
The liquefaction model accounts for many geologic features that strain the prevailing evolutionary models. The vast areas covered by sedimentary layers of extremely uniform thickness and high purity is best described in terms of liquefaction, Some features that would appear to be inexplicable in terms of modern geologic doctrine are predicted in the liquefaction model (e.g., the absence of meteorites in deep sediments is consistent only with a rapid deposition of all the sediments in accord with this outline).
Liquefaction and hydroplate theory interlink, inasmuch as the hydroplate model provides raw sediment to sort as a result of the rapid erosion of material east and west of the initial rupture. All the material in the gap between continents shown in Figure 1 became water-borne sediment subjected to waves and tidal action during the flood.
Liquefaction During the Compression Event
While liquefaction operated cyclically throughout the flood phase, it acted massively once during the compression event, at the end of the continental drift phase.
Visualize a deck of cards sliding across the table. Friction from the table acts to slow the bottommost card. That card, in turn, applies a decelerating force on the second card from the bottom. If none of the cards slip, a frictional deceleration force will finally be applied to the top card. But if a lubricant somehow built up between any two cards, the cards above the lubricated layer would not decelerate, but would slide over the decelerating cards below.
Similarly, the decelerating granite hydroplates acted on the bottommost sedimentary layer riding on the hydroplate. Each sedimentary layer, from the bottom to the top, acted in turn to decelerate the topmost layer. As each layer decelerated, it was severely compressed. This is analogous to suddenly squeezing a water-saturated sponge. The sediments were forced into a denser packing arrangement, freeing water in the process. Angular sedimentary particles also broke as they were crushed together. As the broken fragments settled into the water-filled spaces between particles, more water was released. The freed water was then forced up through the sediments, causing massive liquefaction.
As the deceleration (and thus compression) of the sedimentary column increased, the layers became more and more fluid. Eventually, a point could be reached where the sediments were so fluid chat slippage occurred above a given level, as in our deck of cards. Below that level, compression and liquefaction would have been extreme. Fossils below that level would have floated up and collected at this level where sliding took place. This compression event liquefaction era leads to a startling — and significant — result.
The lowest of these levels appears to be the Precambrian-Cambrian interface. The Precambrian, where it exists, is famous for being a thick sedimentary layer containing almost no fossils. Fossils suddenly begin to be found just above the Precambrian-Cambrian interface at the beginning of the Cambrian, Evolutionists interpret the Precambrian as representing 90% of all geologic time — a vast lifeless period, they believe, because fossils are almost never found in Precambrian sediments. Again, the thickness of sedimentary layers is mistakenly associated with passing time.
In the Grand Canyon, the Precambrian-Cambrian interface is an almost flat, horizontal surface that is exposed for 26 miles above the Colorado River. The layers above the interface are generally horizontal, but the layers below are tipped at large angles, and their tipped edges are beveled off horizontally. It appears that, as slippage began during the compression event, the layers below the slippage plane continued to compress to the point where they buckled. The sliding sedimentary block above the slippage plane beveled off the layers that were being increasingly tipped. See Figure 11.
The conjunction of the hydroplate theory’s compression event with the phenomenon of liquefaction offers a clear explanation for the virtual absence of fossils in the world’s so-called Precambrian geological layers. Liquefaction as it was driven by globe-encircling, self-reinforcing tidal waves prior to the receding of the waters, operating twice a day over a sufficient period of time, effected a high level of sedimentary sorting and fossil sorting. The causes proposed by this model account for the many effects seeking explication. Although the theory is by no means complete, it appears to have met the initial evaluative criteria better than its evolutionary counterparts. Where it differs from prevailing creationist geology, it is hoped that it has done so justifiably, in the interest of a better handling of both the Scriptural and scientific data. The author acknowledges a debt to the many pioneering creationists who’ve gone before, and who continue to develop the implications of this field.
Limitations of this Condensation
In this short space, not every detail could be elaborated. Fuller explanations, with detailed technical notes, are to be found in the source volume. In The Beginning. Some topics warrant entire chapters in themselves (e.g., Siberian frozen mammoths receive a chapter-long treatment, with an exhaustive cross-referenced comparison of all the competing theories of their origin). The volume includes a compendium of creation-oriented ammunition on many topics. The hydroplate theory constitutes the second of three major subdivisions of the work. Christians serious about creation and the flood would do well to add this volume to their libraries. The Center for Scientific Creation markets a videotape, God’s Power & Scripture’s Authority, that covers the topics mentioned in this article.
The Hydroplate Theory and the Scriptures
The ultimate court of appeal for any theory remains the Holy Bible. How does the hydroplate theory stand when summoned before Its bar? Does it reflect scriptural teaching? Does it do so better than the well-known interpretations with which we’ve become accustomed over the years? This, more than the theory’s accord with the scientific evidence, is the pivotal matter to be judged.
Scripture appears to support the contention that there were large quantities of subterranean water in the ancient past. “He has founded it [the earth] upon the seas...” (Ps. 24:2) “He gathers the waters of the sea together as a heap; He lays up the deeps in storehouses...” (Ps. 33:7 — a storehouse is a closed container, possibly the interconnected chambers of the hydroplate theory.) “He lays the beams of His upper chambers in the waters...” (Ps. 104:3) “He spread out the earth above the waters...” (Ps. 136:6) “The earth was formed out of water and by water.” (II Peter 3:5).
These subterranean waters burst forth bringing on the flood, “...the fountains of the great deep burst open, and the floodgates of the sky were opened. And rain fell...” (Cen. 7:11-12 — the sequence of these two events [the bursting open of the fountains of the great deep, and the opening of the floodgates of the sky] is in cause-and-effect order in the hydroplate theory, in parallel with Cen. 8:2 and Prov. 3:20.) “...the sea...bursting forth, it went out from the womb; when I made a cloud its garment....” (Job 38:4-11) “...the channels of water appeared, and the foundations of the world were laid bare...” (Ps. 18:15) “...the deeps were broken up and the ski dripped dew...” (Prov. 3:20).
After a time, the avalanche of water ceased, but the waters continued to rise. “And the rain fell upon the earth for forty days and forty nights.” (Cen. 7:12 — the terra for rain is not the one used for normal rain, matar, but rather geshem, the most violent and deadly rain, in keeping with the violence of the floodgate terminology and the violent bursting open of the fountains of the great deep.) “And the water prevailed upon the earth one hundred and fifty days...and at the end of one hundred and fifty days the water decreased.” (Cen. 7:24, 8:3 — the rain ended after 40 days, but the floodgates weren’t closed until 150 days had passed and the waters had covered the highest mountains.)
Mountains dramatically formed as the flood waters receded, “...the waters were standing above the mountains. At Thy rebuke they fled; at the sound of Thy thunder they hurried away. The mountains rose; the valleys sank down to the place which Thou didst establish for them. Thou didst set a boundary that they [the water] may not pass over; that they may not return to cover the earth.” (Ps. 104:5-9 — God, by raising the mountains and draining the water into enormous basins, thereby created a boundary that the waters could never again pass over. The sound of His thunder may correspond to the ear-shattering sounds attending the compression event and the violent creation of the mountain ranges from the decelerating hydroplates, although this association is speculative.)
Some subterranean water still remains, cf. “...the water under the earth” (Fx. 20:4). Continent-sized plates, settling onto the floor of the subterranean chamber, would trap water in the irregularities at the interface. Such trapped water under continents seems to explain mysteries associated with shallow earthquakes and why deep drilling has intersected “hot flowing water” that is too deep to have seeped down from the earth’s surface.
Dr. Walt Brown and the Center for Scientific Creation
Clearly, only a tiny portion of Dr. Brown’s creationist magnum opus could be presented here. The original book is accessible to both lay people and technical readers (who will spend much of their time poring over the extensive notes sections and technical appendices).
Chalcedon supports the continued application of the Scripture to every scientific discipline, including historical geology. Dr. Brown’s insights are fresh and provocative. An able debater and lecturer, he can be reached at http://www.creationscience.com. Write him care of CSC at 5612 N. 20th Place, Phoenix, AZ 85016 USA.
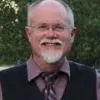
- Martin G. Selbrede
Martin is the senior researcher for Chalcedon’s ongoing work of Christian scholarship, along with being the senior editor for Chalcedon’s publications, Arise & Build and The Chalcedon Report. He is considered a foremost expert in the thinking of R.J. Rushdoony. A sought-after speaker, Martin travels extensively and lectures on behalf of Christian Reconstruction and the Chalcedon Foundation. He is also an accomplished musician and composer.